Abstract
The lens represents an ideal model system for studying many of the cellular and molecular events of differentiation. It is composed of two ectodermally-derived cell types: the lens epithelial cells and the lens fibre cells, which are derived from the lens epithelial cells by differentiation. Programmed removal of nuclei and other organelles from the lens fibre cells ensures that an optically clear structure is created, while the morphology of the degenerating nuclei is similar to that observed during apoptosis and is accompanied by DNA fragmentation. These observations suggest the existence of biochemical parallels between the process of lens fibre cell organelle loss and classical apoptosis. For example, proteins encoded by the bcl-2 and caspase gene families are expressed in developing lenses and nuclear degeneration in lens fibre cells can be inhibited in vivo by overexpression of bcl-2 and in vitro by incubation of differentiating lens epithelial cell cultures with caspase inhibitors. Thus, the developing lens may represent a particularly useful model system for researchers interested in apoptosis. In this review, the recent literature pertaining to lens fibre cell organelle loss and its relationship to apoptosis is reviewed and possible future research directions are suggested.
Similar content being viewed by others
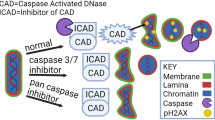
References
Grainger RM. Embryonic lens induction: shedding light on vertebrate tissue determination. Trends Genet 1992; 8: 349–355.
McAvoy JW, Chamberlain CG, de Iongh RU, Hales AM, Lovicu FJ. Lens development. Eye 1999; 13: 425–437.
Piatigorsky J. Lens differentiation in vertebrates. A review of cellular and molecular features. Differentiation 1981; 19: 134–153.
Bassnett S. The fate of the Golgi apparatus and the endoplasmic reticulum during lens fiber cell differentiation. Invest Ophthalmol Vis Sci 1995; 36: 1793–1803.
Potts JD, Bassnett S, Beebe DC. Expression of transforming growth factor beta in the embryonic avian lens coincides with the presence of mitochondria. Dev Dyn 1995; 203: 317–323.
Wride MA. Cellular and molecular features of lens differentiation: A review of recent advances. Differentiation 1996; 61: 77–93.
Bassnett S, Mataic D. Chromatin degradation in differentiating fiber cells of the eye lens. J Cell Biol 1997; 137: 37–49.
Wride MA, Sanders EJ. Nuclear degeneration in the developing lens and its regulation by TNFalpha. Exp Eye Res 1998; 66: 371–383.
Appleby DW, Modak SP. DNA degradation in terminally differentiating lens fiber cells from chick embryos. Proc Natl Acad Sci USA 1977; 74: 5579–5583.
Lang RA. Apoptosis in mammalian eye development: Lens morphogenesis, vascular regression, and immue privilege. Cell Death & Differ 1997; 4: 12–20.
Counis MF, Chaudun E, Arruti C, et al. Analysis of nuclear degradation during lens cell differentiation. Cell Death Differ 1998; 5: 251–261.
DahmR. Lens fibre cell differentiation-A link with apoptosis? Ophthalmic Res 1999; 31: 163–183.
Bassnett S, Beebe DC. Coincident loss of mitochondria and nuclei during lens fiber cell differentiation. Dev Dyn 1992; 194: 85–93.
Dahm R, Gribbon C, Quinlan RA, Prescott AR. Changes in the nucleolar and coiled body compartments precede lamina and chromatin reorganization during fibre cell denucleation in the bovine lens. Eur J Cell Biol 1998; 75: 237–246.
Bassnett S. Fiber cell denucleation in the primate lens. Invest Ophthalmol Vis Sci 1997; 38: 1678–1687.
McAvoy JW, Richardson NA. Nuclear pyknosis during lens fibre differentiation in epithelial explants. Curr Eye Res 1986; 5: 711–715.
Chaudun E, Arruti C, Courtois Y, et al. DNA strand breakage during physiological apoptosis of the embryonic chick lens: Free 30 OH end single strand breaks do not accumulate even in the presence of a cation-independent deoxyribonuclease.J Cell Physiol 1994; 158: 354–364.
Wride MA, Parker E, Sanders EJ. Members of the bcl-2 and caspase families regulate nuclear degeneration during chick lens fibre differentiation. Dev Biol 1999; 213: 142–156.
Zimmerman LE, Font RL. Congenital malformations of the eye. JAMA 1966; 196: 96–104.
Dahm R, Gribbon C, Quinlan RA, Prescott AR. Susceptibility of lens epithelial and fibre cells at different stages of differentiation to apoptosis. Biochem Soc Trans 1998; 26: S349.
Vrensen GF, Graw J, De Wolf A. Nuclear breakdown during terminal differentiation of primary lens fibres in mice: A transmission electron microscopic study. Exp Eye Res 1991; 52: 647–659.
He HY, Gao C, Vrensen G, Zelenka P. Transient activation of cyclin B/Cdc2 during terminal differentiation of lens fiber cells. Dev Dyn 1998; 211: 26–34.
Xue SP, Zhang SF, Du Q, et al. The role of cytoskeletal elements in the two-phase denucleation process of mammalian erythroblasts in vitro observed by laser confocal scanning microscope. Cell Mol Biol 1997; 43: 851–860.
Capetanaki Y, Smith S, Heath JP. Overexpression of the vimentin gene in transgenic mice inhibits normal lens cell differentiation. J Cell Biol 1989; 109: 1653–1664.
Lovicu FJ, McAvoy JW. Spatial and temporal expression of p57(KIP2) during murine lens development. Mech Dev 1999; 86: 165–169.
Gao CY, Rampalli AM, Cai HC, He HY, Zelenka PS. Changes in cyclin dependent kinase expression and activity accompanying lens fiber cell differentiation. Exp Eye Res 1999; 69: 695–703.
Kroemer G. The proto-oncogene Bcl-2 and its role in regulating apoptosis. Nat Med 1997; 3: 614–620.
Adams JM, Cory S. The Bcl-2 protein family: Arbiters of cell survival. Science 1998; 281: 1322–1326.
Green DR, Reed JC. Mitochondria and apoptosis. Science 1998; 2811309–1312.
ChaoDT, Korsmeyer SJ. Bcl-2 family: Regulators of cell death. Annu Rev Immunol 1998; 16: 395–419.
Fromm L, Overbeek PA. Inhibition of cell death by lens-specific overexpression of bcl-2 in transgenic mice. Dev Genet 1997; 20: 276–287.
Eskes R, Desagher S, Antonsson B, Martinou JC. Bid induces the oligomerization and insertion of bax into the outer mitochondrial membrane. Mol Cell Biol 2000; 20: 929–935.
Schurmann A, Mooney AF, Sanders LC, et al. p21-activated kinase 1 phosphorylates the death agonist bad and protects cells from apoptosis. Mol Cell Biol 2000; 20: 453–461.
Scheid MP, Schubert KM, Duronio V. Regulation of bad phosphorylation and association with Bcl-x(L) by the MAPK/Erk kinase. J Biol Chem 1999; 274: 31108–31113.
Porter AG. Protein translocation in apoptosis. Trends Cell Biol 1999; 9: 394–401.
Jiang JX, Goodenough DA. Retroviral expression of connexins in embryonic chick lens. Invest Ophthalmol Vis Sci 1998; 39: 537–543.
Alnemri ES, Livingston DJ, Nicholson DW, et al. Human ICE/CED-3 protease nomenclature. 1996; 87: 171.
Cryns V, Yuan J. Proteases to die for. Genes Dev 1998; 12: 1551–1570.
Budihardjo I, Oliver H, Lutter M, Luo X, Wang X. Biochemical pathways of caspase activation during apoptosis. Annu Rev Cell Dev Biol 1999; 15: 269–290.
Kidd VJ. Proteolytic activities that mediate apoptosis. Annu Rev Physiol 1998; 60: 533–573.
Duriez PJ, Shah GM. Cleavage of poly(ADP-ribose) polymerase: A sensitive parameter to study cell death. Biochem Cell Biol 1997; 75: 337–349.
Cohen GM. Caspases: The executioners of apoptosis. Biochem J 1997; 326 (Pt 1): 1–16.
Ishizaki Y, Jacobson MD, Raff MC. A role for caspases in lens fiber differentiation. J Cell Biol 1998; 140: 153–158.
Rao L, Perez D, White E. Lamin proteolysis facilitates nuclear events during apoptosis. J Cell Biol 1996; 135: 1441–1455.
Bernardi P, Scorrano L, Colonna R, Petronilli V, Di Lisa F. Mitochondria and cell death. Mechanistic aspects and methodological issues. Eur J Biochem 1999; 264: 687–701.
Bassnett S. Mitochondrial dynamics in differentiating fiber cells of the mammalian lens. Curr Eye Res 1992; 11: 1227–1232.
Lorenzo HK, Susin SA, Penninger J, Kroemer G. Apoptosis inducing factor (AIF): A phylogenetically old, caspaseindependent effector of cell death. Cell Death Differ 1999; 6: 516–524.
van Leyen K, Duvoisin RM, Engelhardt H, Wiedmann M. A function for lipoxygenase in programmed organelle degradation. Nature 1998; 395: 392–395.
Rath PC, Aggarwal BBTNF-induced signaling in apoptosis. J Clin Immunol 1999; 19: 350–364.
Abbadie C, Kabrun N, Bouali F, et al. High levels of c-rel expression are associated with programmed cell death in the developing avian embryo and in bone marrow cells in vitro. Cell 1993; 75: 899–912.
Cecconi F, Alvarez-Bolado G, Meyer BI, Roth KA, Gruss P. Apaf1 (CED-4 homolog) regulates programmed cell death in mammalian development. Cell 1998; 94: 727–737.
Pan H, Griep AE. Altered cell cycle regulation in the lens of HPV-16 E6 or E7 transgenic mice: Implications for tumor suppressor gene function in development. Genes Dev 1994; 8: 1285–1299.
Pan H, Griep AE. Temporally distinct patterns of p53-dependent and p53-independent apoptosis during mouse lens development. Genes Dev 1995; 9: 2157–2169.
Stolen CM, Griep AE. Disruption of lens ffiber cell differentiation and survival at multiple stages by region-specific expression of truncated FGF receptors. Dev Biol 2000; 217: 205–220.
Liegeois NJ, Horner JW, DePinho RA. Lens complementation system for the genetic analysis of growth, differentiation, and apoptosis in vivo. Proc Natl Acad Sci USA 1996; 93: 1303–1307.
Author information
Authors and Affiliations
Rights and permissions
About this article
Cite this article
Wride, M.A. Minireview: Apoptosis as seen through a lens. Apoptosis 5, 203–209 (2000). https://doi.org/10.1023/A:1009653326511
Issue Date:
DOI: https://doi.org/10.1023/A:1009653326511